[AWC 3rd Place] Rewriting Destiny: Genetic Engineering's Potential in P. gingivalis-Associated AD
1.0 Introduction
Genetic engineering, a cutting-edge field of biotechnology, has opened up new frontiers in medical research and treatment (Komor et al., 2016; Shin et al., 2017; Lee et al., 2019). One of the most intriguing areas of study is the potential link between genetic engineering and Alzheimer's Disease (AD), a neurodegenerative disorder characterized by cognitive decline and memory loss (Bahar et al., 2021). Emerging research has unveiled a connection between the oral pathogen Porphyromonas gingivalis (P. gingivalis) and AD (Dominy et al., 2019). As the scientific community peers into this uncharted territory, an enthralling narrative emerges at the juncture of genetic engineering, P. gingivalis, and AD, hinting at the transformative potential of genetic manipulation in deepening our comprehension and redefining our approach to combatting this complex malady. This article delves into the intersection of genetic engineering, P. gingivalis, and AD, shedding light on the potential for genetic manipulation to revolutionize our understanding and management of this debilitating disease.
1.1 P gingivalis & AD
AD, an intricate mosaic of neuronal dysfunction, has long posed one of the most profound challenges in modern medicine (Association, 2019). Characterized by the insidious degradation of cognitive abilities, memory loss, and emotional upheavals, AD imposes an immense burden on patients, caregivers, and healthcare systems worldwide (Atri, 2019). Yet, as researchers strive to decipher its underlying mechanisms, they have encountered a compelling clue – an unexpected and intricate web of interactions between AD and an entity far removed from the cerebral realm: Porphyromonas gingivalis.
Traditionally known as an oral pathogen, P. gingivalis's association with AD has ignited a paradigm shift in how we perceive the intricate interplay between oral health and cognitive well-being (Bostanci and Belibasakis, 2012). Emerging studies have illuminated a link between chronic periodontal infections, often instigated by P. gingivalis, and a heightened susceptibility to AD. This connection appears to stem from a cascade of events wherein P. gingivalis, originating in the oral cavity, disseminates and potentially triggers neuroinflammation and the accumulation of amyloid beta plaques in the brain – hallmark features of AD pathology (Dominy et al., 2019; Patel et al., 2021).
In the midst of this scientific intrigue, genetic engineering emerges as a formidable vanguard, poised to reshape our understanding of this intricate web of connections. This innovative discipline enables scientists to not merely observe from a distance but to actively delve into the genetic codes that govern both P. gingivalis and AD. Through precise manipulation of genetic material, scientists can craft bespoke models that mirror the interactions between the pathogen and the brain, illuminating pathways, interactions, and potential causal relationships.
2.0 Genetic Engineering: A Tool for Precision Investigation and Treatment
Genetic engineering offers a powerful toolset to explore the complex relationship between P. gingivalis and AD. With techniques like CRISPR-Cas9, scientists can engineer animal models that mimic the interactions between the bacteria and the brain, allowing for a more detailed understanding of the mechanisms at play (Shin et al., 2017). Moreover, genetic manipulation enables researchers to modify specific genes associated with both P. gingivalis infection and AD pathology, offering insights into causality and potential therapeutic targets (Visanji et al., 2015).
For example:
Creating Animal Models to Mimic P. gingivalis Interactions
Genetic engineering allows scientists to engineer animal models that closely mimic the interactions between P. gingivalis and the brain. By introducing specific genetic modifications to replicate the inflammatory response seen in chronic periodontal infections, researchers can observe the progression of AD-like pathology and gain insights into the causal relationship between P. gingivalis and AD. These models help identify key molecular players and pathways, aiding in the development of targeted therapies. Similar study done Phillips et al., has shown promising outcomes for cell transplantation using these modifications (Phillips and Tang, 2008).
Gene Editing to Unravel Mechanisms
CRISPR-Cas9 technology has enabled scientists to edit specific genes involved in P. gingivalis infection and AD pathology. For example, researchers can manipulate genes responsible for amyloid beta production or immune response regulation. By observing the effects of these genetic modifications, scientists gain a deeper understanding of how P. gingivalis contributes to amyloid plaque formation and neuroinflammation, shedding light on potential therapeutic targets.
Exploring Genetic Susceptibility
Genetic engineering allows researchers to identify and manipulate genes associated with susceptibility to both P. gingivalis infection and AD. By studying individuals with genetic predispositions to periodontal diseases and AD, scientists can pinpoint shared genetic factors that contribute to susceptibility. For instance, variations in immune response genes might influence the likelihood of developing both conditions. Such insights guide the development of personalized preventive strategies and therapies tailored to an individual's genetic makeup.
Editing Immune Response Genes:
In a breakthrough study, researchers used CRISPR-Cas9 to modify immune response genes in a mouse model susceptible to P. gingivalis infection (Solbiati et al., 2020). By enhancing the mice's immune system to better combat the pathogen, they observed a significant reduction in both oral bacterial load and brain inflammation. This success demonstrates the potential of genetic engineering to strengthen the body's defenses against P. gingivalis, potentially slowing the progression of AD.
Alleviating Neuroinflammation:
Genetic engineering holds promise for designing therapies that target neuroinflammation triggered by P. gingivalis. By manipulating genes responsible for regulating immune responses in the brain, scientists can potentially develop treatments that suppress excessive inflammation while preserving essential immune functions. This approach has the potential to mitigate the neurodegenerative effects of chronic infections and contribute to AD management.
Reducing Amyloid Beta Accumulation:
In another notable study, researchers used gene editing techniques to modify genes associated with amyloid beta production in a mouse model (Lu et al., 2021). By reducing the expression of these genes, they observed a decrease in amyloid beta plaque accumulation in the brain. This success exemplifies how genetic engineering can directly impact AD pathology by altering the molecular processes that lead to plaque formation.
3.0 Exploring Genetic Susceptibility
In the intricate tapestry of human health, genetics plays a fundamental role in determining susceptibility to various diseases (The genetic basis of disease - PMC, no date). Among the intriguing frontiers of research lies the exploration of genetic susceptibility to both P. gingivalis infection and AD. By delving into the genetic makeup of individuals who are predisposed to these conditions, scientists aim to unearth the hidden threads that connect these seemingly distinct entities. This quest for genetic susceptibility not only enhances our understanding of disease mechanisms but also holds the potential to revolutionize preventive strategies and therapeutic interventions.
Unraveling the Genetic Blueprint of P. gingivalis-Associated AD
In the intricate tapestry of human health, genetics plays a fundamental role in determining susceptibility to various diseases. Among the intriguing frontiers of research lies the exploration of genetic susceptibility to both P. gingivalis infection and AD. By delving into the genetic makeup of individuals who are predisposed to these conditions, scientists aim to unearth the hidden threads that connect these seemingly distinct entities. This quest for genetic susceptibility not only enhances our understanding of disease mechanisms but also holds the potential to revolutionize preventive strategies and therapeutic interventions.
In our very own discovery using bioinformatics we have discovered the common genes associated with P gingivalis and AD, paving the early stages of a genetic predisposition to Pg-AD association (Figure 1) (Hamarsha et al., 2023).
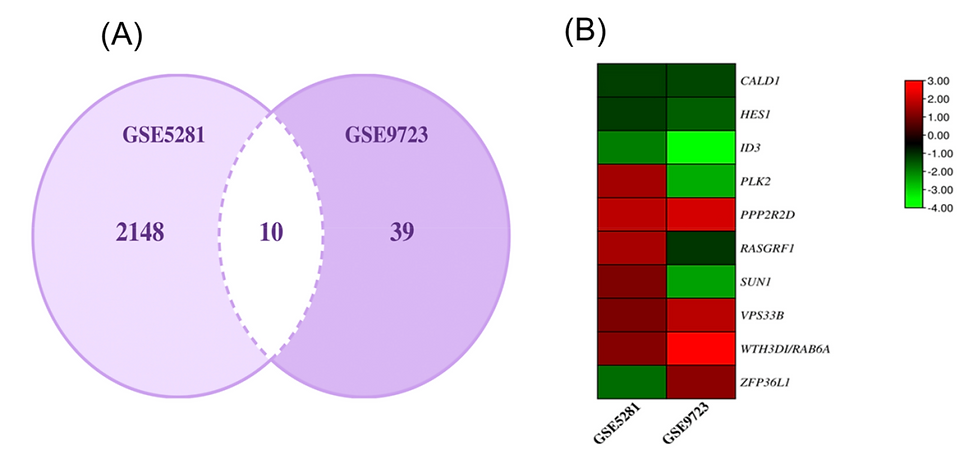
Figure 1 Common genes identified through GEO and bioinformatic tools in our study (Hamarsha et al., 2023).
The Genetic Landscape: Predisposition to P. gingivalis and AD
Human genetics are a mosaic of intricate variations, with each individual carrying a unique set of genetic instructions that influence their susceptibility to diseases. Researchers have begun to unravel the genetic signatures associated with both chronic periodontal infections caused by P. gingivalis and the development of AD. Through large-scale genetic studies, scientists have identified specific genetic markers that are more prevalent in individuals with these conditions, hinting at shared underlying vulnerabilities.
Unmasking Shared Genetic Factors: Insights from Research
By comparing the genetic profiles of individuals with periodontal diseases and those with AD, researchers have unearthed intriguing overlaps in susceptibility genes. These shared genetic factors may encompass elements that regulate immune responses, inflammation, and neuronal health. For instance, variations in genes associated with the immune system's ability to recognize and combat P. gingivalis could concurrently influence the inflammatory response in the brain—a hallmark of AD pathology.
The Promise of Personalized Prevention: Tailoring Strategies for Individuals
The revelation of common genetic factors linking P. gingivalis susceptibility and AD opens the door to personalized prevention strategies. Armed with the knowledge of specific genetic markers, healthcare practitioners could identify individuals at higher risk for P. gingivalis-related AD early in life. Such insights enable the development of tailored interventions, including targeted oral hygiene regimens, dietary modifications, and lifestyle adjustments, to mitigate both chronic periodontal infections and neurodegenerative processes.
Genetic Interventions: Rewriting Susceptibility Profiles
The convergence of genetics and technology paves the way for innovative genetic interventions (Genomic translational research: Paving the way to individualized cardiac functional analyses and personalized cardiology - PMC, no date). As our understanding of the genetic basis of P. gingivalis-associated AD deepens, scientists could envision therapies aimed at modifying susceptibility genes. By leveraging advanced genetic engineering techniques, researchers might enhance an individual's immune response against P. gingivalis, bolster neuronal protection mechanisms, or alter molecular pathways that contribute to AD progression. Such interventions, administered with precision, have the potential to rewrite an individual's susceptibility profile and significantly reduce the risk of developing P. gingivalis-related AD.
4.0 Targeted Therapies and Preventive Interventions
Genetic engineering also holds promise for developing novel treatments for P. gingivalis-associated AD. By manipulating genes involved in immune responses or neuronal protection, scientists may design therapies aimed at reducing the impact of chronic infections and the subsequent neuroinflammation. Furthermore, the ability to edit the genome could lead to innovative preventive interventions, such as altering the expression of genes responsible for producing amyloid beta plaques, thereby slowing the progression of AD.
One of the cornerstones of genetic engineering's potential lies in its ability to manipulate genes that govern the immune response. Neuroinflammation, a hallmark of AD progression triggered by P. gingivalis, inflicts significant damage on brain cells. Genetic engineers can craft therapeutic interventions that bolster the immune system's ability to recognize and combat the pathogen, attenuating the inflammatory cascade. By precisely enhancing immune response genes, researchers envision therapies that could curtail the impact of chronic infections and quell the neuroinflammation storm that contributes to cognitive decline.
Another promising avenue in the genetic engineering arsenal involves fortifying neuronal protection mechanisms. In AD, the integrity of neurons is compromised, leading to cognitive impairment. Genetic manipulation holds the potential to enhance genes responsible for neuronal resilience and survival. By modifying these genes, scientists could craft therapies that fortify brain cells, rendering them more resistant to the ravages of P. gingivalis-induced neurodegeneration. This approach not only addresses the symptoms but also targets the core mechanisms driving cognitive decline.
Genetic engineering's potential extends beyond treatment into the realm of prevention. As scientists amass insights into the genetic architecture of P. gingivalis-associated AD, they can envisage innovative strategies to alter the course of the disease before symptoms manifest. The ability to edit the genome offers a tantalizing prospect: modifying the expression of genes that contribute to the production of amyloid beta plaques – the pathological culprits underpinning AD. By curbing their overproduction or enhancing their clearance, researchers envision a potential avenue to slow the progression of AD, heralding a paradigm shift in preventive interventions.
Genetic engineering's precision allows for the tailoring of therapies to individual genetic profiles. Through comprehensive genetic analysis, healthcare practitioners could identify genetic susceptibility factors that predispose individuals to P. gingivalis-associated AD. Armed with this knowledge, personalized treatments could be developed, targeting specific genetic pathways to optimize therapeutic outcomes. This approach aligns with the overarching goal of precision medicine – to deliver interventions that are finely attuned to each patient's unique genetic makeup.
5.0 Ethical and Social Considerations
The rapid advancement of genetic engineering has ushered in a new era of possibilities in medicine and biotechnology. However, along with the tremendous potential come a myriad of ethical and social considerations that demand thoughtful deliberation and careful navigation. These considerations revolve around ensuring that genetic engineering is used in a responsible, equitable, and morally sound manner, preserving fundamental values while harnessing the benefits of scientific progress (Risks et al., 1994).
5.1 Responsible Use of Genetic Interventions
As the power of genetic engineering grows, the ethical question arises: what should we modify and why? The ability to alter genes raises concerns about the distinction between therapy and enhancement (Moving Beyond ‘Therapy’ and ‘Enhancement’ in the Ethics of Gene Editing | Cambridge Quarterly of Healthcare Ethics | Cambridge Core, no date). While genetic interventions might hold the promise of preventing or treating diseases, there's a risk of venturing into territory where we are modifying non-disease traits for cosmetic or enhancement purposes. Ethical guidelines are needed to delineate the boundaries of acceptable genetic modifications, keeping the focus on addressing medical needs and avoiding unnecessary alterations.
5.2 Unintended Consequences and Long-Term Effects
Genetic engineering, like any scientific advancement, carries the potential for unintended consequences (Health, 2004). Manipulating genes can lead to unforeseen outcomes that may not manifest until years or even generations later (Rubeis and Steger, 2018). These unintended effects could impact not only the individuals who undergo genetic interventions but also their descendants. Ethical considerations encompass the duty to thoroughly research, predict, and mitigate potential risks before introducing genetic modifications into humans.
5.3 Equitable Access and Social Justice
One of the foremost ethical challenges is ensuring equitable access to genetic interventions (Jooma et al., no date). Cutting-edge therapies can be expensive to develop and implement, potentially leading to disparities in healthcare access. It's crucial to avoid exacerbating existing social inequalities by creating a divide between those who can afford genetic interventions and those who cannot. Ethical frameworks must prioritize accessibility, affordability, and fair distribution of genetic therapies to prevent further marginalization of vulnerable populations (What Makes Clinical Research in Developing Countries Ethical? The Benchmarks of Ethical Research | The Journal of Infectious Diseases | Oxford Academic, no date).
5.4 Informed Consent and Autonomy
Genetic engineering involves altering an individual's fundamental biological makeup, making informed consent a critical ethical consideration (Ormandy, Dale and Griffin, 2011). Individuals must be fully informed about the potential risks, benefits, and uncertainties associated with genetic interventions. This empowers them to make autonomous decisions about their own bodies and genetic makeup. Respecting autonomy and ensuring that consent is genuinely informed, and voluntary is central to upholding ethical standards.
5.5 Environmental and Ecological Impact
The repercussions of genetic engineering extend beyond individual human health and can impact ecosystems and the environment. For example, gene-edited organisms released into the wild could potentially disrupt ecosystems or introduce unintended consequences (Ormandy, Dale and Griffin, 2011). Ethical discussions need to encompass the broader ecological implications of genetic interventions and consider their potential impact on biodiversity and ecosystems.
5.6 Cultural and Religious Considerations
Genetic engineering raises cultural and religious concerns that vary across different societies. Beliefs about the sanctity of life, the role of humans in altering nature, and the definition of normality can shape attitudes towards genetic interventions (Weingarten, 2007). Ethical frameworks should accommodate diverse perspectives and engage in respectful dialogue to ensure that scientific advancements align with cultural and religious values.
5.7 Balancing Progress and Ethics
The march of genetic engineering holds the promise of transformative breakthroughs in healthcare and beyond. However, its responsible advancement hinges on an unwavering commitment to ethical principles. Striking a balance between scientific progress and ethical frameworks is essential to ensure that genetic engineering benefits humanity without compromising human rights, values, and the well-being of present and future generations. Open, transparent, and inclusive discussions involving scientists, ethicists, policymakers, and the broader public are essential to guide the ethical evolution of this powerful technology.
6.0 Conclusions
Genetic engineering offers an unprecedented opportunity to unravel the intricate relationship between P. gingivalis infection and AD. By leveraging advanced genetic manipulation techniques, researchers can uncover crucial insights into disease mechanisms, susceptibility factors, and therapeutic avenues. While ethical and societal considerations must guide the application of genetic engineering in humans, the potential to mitigate the impact of P. gingivalis-associated AD marks a significant stride towards understanding and treating this devastating condition.
References
Association, A. (2019) ‘2019 Alzheimer’s disease facts and figures’, Alzheimer’s & dementia,15(3), pp. 321–387.
Atri, A. (2019) ‘The Alzheimer’s Disease Clinical Spectrum: Diagnosis and Management’, The Medical clinics of North America, 103(2), pp. 263–293. Available at: https://doi.org/10.1016/J.MCNA.2018.10.009.
Bahar, B. et al. (2021) ‘Porphyromonas gingivalis (W83) infection induces alzheimer’s disease-like pathophysiology in obese and diabetic mice’, Journal of Alzheimer’s Disease, 82(3), pp. 1259–1275. Available at: https://doi.org/10.3233/JAD-210465.
Bostanci, N. and Belibasakis, G.N. (2012) ‘Porphyromonas gingivalis: an invasive and evasive opportunistic oral pathogen’, FEMS microbiology letters, 333(1), pp. 1–9. Available at: https://doi.org/10.1111/J.1574-6968.2012.02579.X.
Dominy, S.S. et al. (2019) ‘Porphyromonas gingivalis in Alzheimer’s disease brains: Evidence for disease causation and treatment with small-molecule inhibitors’, Science Advances, 5(1). Available at: https://doi.org/10.1126/SCIADV.AAU3333.
Genomic translational research: Paving the way to individualized cardiac functional analyses and personalized cardiology - PMC (no date). Available at: https://www.ncbi.nlm.nih.gov/pmc/articles/PMC5332127/ (Accessed: 12 August 2023).
Hamarsha, A. et al. (2023) ‘Predicting Key Genes and Therapeutic Molecular Modelling to Explain the Association between Porphyromonas gingivalis (P. gingivalis) and Alzheimer’s Disease (AD)’, International Journal of Molecular Sciences, 24(6), p. 5432. Available at: https://doi.org/10.3390/ijms24065432.
Health, N.R.C. (US) C. on I. and A.U.E. of G.E.F. on H. (2004) ‘Unintended Effects from Breeding’, in Safety of Genetically Engineered Foods: Approaches to Assessing Unintended Health Effects. National Academies Press (US). Available at: https://www.ncbi.nlm.nih.gov/books/NBK215778/ (Accessed: 12 August 2023).
Jooma, S. et al. (no date) ‘Defining and Achieving Health Equity in Genomic Medicine’, Ethnicity & Disease, 29(Suppl 1), pp. 173–178. Available at: https://doi.org/10.18865/ed.29.S1.173.
Komor, A.C. et al. (2016) ‘Programmable editing of a target base in genomic DNA without double-stranded DNA cleavage’, Nature, 533(7603), pp. 420–424. Available at: https://doi.org/10.1038/nature17946.
Lee, H.K. et al. (2019) ‘Simultaneous targeting of linked loci in mouse embryos using base editing’, Scientific Reports, 9(1), p. 1662. Available at: https://doi.org/10.1038/s41598-018-33533-5.
Lu, L. et al. (2021) ‘Application of CRISPR/Cas9 in Alzheimer’s Disease’, Frontiers in Neuroscience, 15, p. 803894. Available at: https://doi.org/10.3389/fnins.2021.803894.
Moving Beyond ‘Therapy’ and ‘Enhancement’ in the Ethics of Gene Editing | Cambridge Quarterly of Healthcare Ethics | Cambridge Core (no date). Available at: https://www.cambridge.org/core/journals/cambridge-quarterly-of-healthcare-ethics/article/abs/moving-beyond-therapy-and-enhancement-in-the-ethics-of-gene-editing/0C3C87FF1344EEC69D5457A4E52B58C8 (Accessed: 12 August 2023).
Ormandy, E.H., Dale, J. and Griffin, G. (2011) ‘Genetic engineering of animals: Ethical issues, including welfare concerns’, The Canadian Veterinary Journal, 52(5), pp. 544–550.
Patel, S. et al. (2021) ‘Characterization of Human Genes Modulated by Porphyromonas gingivalis Highlights the Ribosome, Hypothalamus, and Cholinergic Neurons’, Frontiers in Immunology, 12, p. 646259. Available at: https://doi.org/10.3389/fimmu.2021.646259.
Phillips, M.I. and Tang, Y.L. (2008) ‘Genetic Modification of Stem Cells for Transplantation’, Advanced drug delivery reviews, 60(2), pp. 160–172. Available at: https://doi.org/10.1016/j.addr.2007.08.035.
Risks, I. of M. (US) C. on A.G. et al. (1994) ‘Social, Legal, and Ethical Implications of Genetic Testing’, in Assessing Genetic Risks: Implications for Health and Social Policy. National Academies Press (US). Available at: https://www.ncbi.nlm.nih.gov/books/NBK236044/ (Accessed: 12 August 2023).
Rubeis, G. and Steger, F. (2018) ‘Risks and benefits of human germline genome editing: An ethical analysis’, Asian Bioethics Review, 10(2), pp. 133–141. Available at: https://doi.org/10.1007/s41649-018-0056-x.
Shin, H.Y. et al. (2017) ‘CRISPR/Cas9 targeting events cause complex deletions and insertions at 17 sites in the mouse genome’, Nature Communications, 8(1), p. 15464. Available at: https://doi.org/10.1038/ncomms15464.
Solbiati, J. et al. (2020) ‘Virulence of the Pathogen Porphyromonas gingivalis Is Controlled by the CRISPR-Cas Protein Cas3’, mSystems, 5(5), pp. e00852-20. Available at: https://doi.org/10.1128/mSystems.00852-20.
The genetic basis of disease - PMC (no date). Available at: https://www.ncbi.nlm.nih.gov/pmc/articles/PMC6279436/ (Accessed: 12 August 2023).
Visanji, N.P. et al. (2015) ‘Deep brain stimulation of the subthalamic nucleus preferentially alters the translational profile of striatopallidal neurons in an animal model of parkinson’s disease’, Frontiers in Cellular Neuroscience, 9(June), pp. 1–11. Available at: https://doi.org/10.3389/FNCEL.2015.00221/ABSTRACT.
Weingarten, M.A. (2007) ‘On the sanctity of life’, The British Journal of General Practice, 57(537), pp. 332–333.
What Makes Clinical Research in Developing Countries Ethical? The Benchmarks of Ethical Research | The Journal of Infectious Diseases | Oxford Academic (no date). Available at: https://academic.oup.com/jid/article/189/5/930/810459 (Accessed: 12 August 2023).
Comments