Sunday Article 07: Genome Editing

Genome Editing: Cheat Code to Gaining Superpowers
In the late 1900s, the first genome editing technologies were invented with tools such as zinc-finger nucleases (ZFNs) and transcription activator-like effector nuclease (TALEN) making it possible for scientists not only to study the effect of mutations in relation to human diseases but also to develop gene therapies in treating diseases.
In 2015, a one-year-old with leukaemia received modified immune cells which replaced the immune cells destroyed by chemotherapy. These modified immune cells were edited using TALEN to disable them from attacking the patient and to enable protection from anti-cancer drugs the patient was taking. This highlights the clinical potential of genome editing and points out the great things we can do with such tools. Then came the CRISPR/Cas9 system which has been a revolution in the scientific world by providing a cheaper and more accurate tool to edit parts of the genome. With the field moving rapidly, questions arise on how far we can go with these technologies.
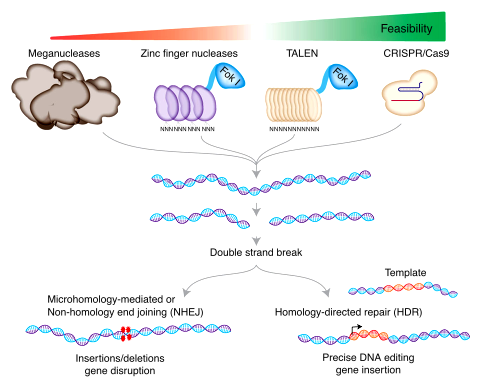
Schematic showing genome editing tools which operate by inducing double-stranded break to edit parts of genes. Source: Mazhar Adli (2018) “The CRISPR tool kit for genome editing and beyond” Nature Communications.
In superhero comics, the concept of ‘enhanced abilities’ is not at all unusual for both superheroes and villains. While some gain their superpowers simply by being non-humans, genetic mutation is also a popular way of getting these abilities. The Marvel comics illustrate this by bringing forward the concept of ‘mutants’-humans possessing genetic trait called the ‘X-gene’ which gives them superpowers (for example most characters in the X-Men franchise) and ‘mutates’- those who are not born with the ‘X-gene’ but acquire superpowers through exposure to mutagenic agents (for example Spider-Man and Captain America).
Since we already have the means to edit human genes, gaining superpowers is plausible, right?
Not quite.
While scientists have shown that genome editing can be done on humans, the focus right now is to improve people’s health and the quality of people’s lives. Not to mention the legality and ethical concerns surrounding human genome editing in the first place. That means superpowers as seen in comic books might still be far out of our reach. However, for the sake of entertaining this idea, the question remains-
How close are we to getting our own superpowers?

Superpower: ‘Pain Tolerance’
SuperAntihero: Deadpool
Pain perception (known as nociception) is often necessary to transmit signals to the brain when there is damage or potential threat to our bodies. Although discomforting, pain provides a mechanism to ensure our survival yet might cause problems when pain shifts from acute to chronic. Chronic pain is prevalent in the global population, affecting 19%-50% of the population. Despite this number, there are still limited treatments available for chronic pain. Non-opioids drugs are often less effective against severe pain while opioid drugs come with the cost of addiction. For cancer patients, chemotherapy often comes with the side-effect of chronic pain and the usage of opioids such as morphine, while allowing patients to tolerate the chemotherapy-associated pain, is also less ideal due to its adverse effects and risk of addiction. Thus, there is a need for treatments that are well-suited for long-term pain management and this can be achieved with genome editing.
Pain can either be nociceptive (arising from actual damage) or neuropathic (arising from damage in the nervous system). Neuropathic pain includes hyperalgesia (abnormal sensitivity to pain) and allodynia (a condition in which pain can occur from non-painful stimuli such as a simple touch). Studies have shown that some of the members in the voltage-gated sodium channel family (NaV) are implicated in pain transmission, including NaV1.7 which is encoded by the gene SCN9A. The gene is often altered in human pain disorders- with the loss-of-function causing pain insensitivity, and the gain-of-function causing extreme pain disorder. With its role in nociception, SCN9A serves as an attractive target for chronic pain treatments. However, small-molecule drugs and antibodies targeting the gene/protein is often ineffective, thus researchers Moreno and colleagues decided to manipulate the expression of NaV1.7 in cells capable of producing neuronal cell types by repressing SCN9A using CRISPR-Cas9. The researchers demonstrated that knockdown of NaV1.7 resulted in a decrease of hyperalgesia. In fact, testing is done on mice which had been administered with a chemotherapy drug, paclitaxel with the side-effect of allodynia also showed a decrease in neuropathic pain. Although the pain was not completely diminished, the significant reduction and the longer-lasting effects showcased the therapeutic potential of genome editing in replacing current treatments for chronic pain, especially for cancer patients undergoing chemotherapy. This, however, is not meant for the permanent alteration of our nociception as pain is still crucial in our normal daily lives.
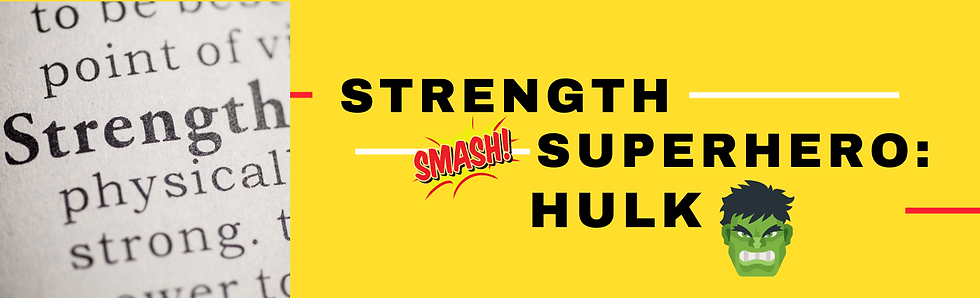
Superpower: ‘Strength’
Superhero: Hulk
Strength often relates to muscle power, with the likes of Hulk and Superman showing superhuman strength when lifting heavy things with ease. In real life, while genome editing tools are not used to enhance strength in humans as seen in Captain America, they have potentials in treating people lacking ‘strength’. This refers to muscle weakness- a common symptom described by a group of conditions known as muscular dystrophies (MDs). Currently, no cures for MDs have been reported but treatments focusing on alleviating the symptoms have been developed. These treatments include disease-modifying therapies which have been used for treating Duchenne muscular dystrophy (DMD)- a monogenic disorder caused by a mutation in the DMD gene on the X-chromosome. One strategy of disease-modifying therapies is to target dystrophin deficiency, but this is still limited in terms of providing long-lived treatment for DMD. Thus, genetic approaches are important to consider especially when the disease itself is caused by a single gene- making it a potential target for gene therapies.
While this is challenging due to the large size of the DMD gene, scientists have demonstrated that correct of the mutation is possible with CRISPR-Cas9. Mutations in the DMD gene often occurs through exon deletion and this results in loss of dystrophin or dysfunctional dystrophin. Referred to as myoediting, one of the strategies in repairing the mutation is by generating a single cut in the gene to induce exon skipping thus avoiding the defective exon. The viral delivery system for myoediting is then used in mice and canine models and it was found that the mutation was corrected alongside the restoration of dystrophin level. While there are still challenges of myoediting such as the off-target activity of CRISPR-Cas system, the success of myoediting in animal models, as well as human induced pluripotent stem cells (iPSCs), shows that it would not be long till myoediting is applied clinically.
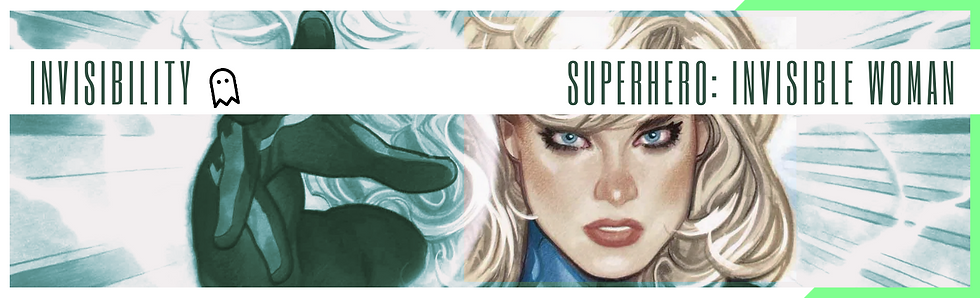
Superpower: ‘Invisibility’
Superhero: Invisible Woman
Most of the times, our immune system is our friend, protecting our bodies from attacks of invaders and pathogens. However, this feature can also lead to our downfall, especially in regards to transplants which are considered as ‘foreign’ by our immune system. As a result, transplant rejection invokes a variety of symptoms from mild flu-like symptoms to organ failure due to chronic rejection. While there are immunosuppressants which can lower the risk of rejection by suppressing our immune system, these leave patients vulnerable to infections as the immune system will not be able to fight them. Scientists then developed iPSCs using patients’ cells to minimise the risk of immune attack. While a great concept, this is still challenging clinically due to cost, time, and the low likelihood for patients’ cells to reprogram into their pluripotent state. To overcome this, Deuse and colleagues then came up with ‘universal’ iPSCs which can be used by all patients. These are engineered using CRISPR/Cas9, creating functionally ‘invisible’ cells which can escape attacks from the immune system.
In the study, major histocompatibility complex (MHC) class I and II genes are targeted so that production of MHC proteins is disrupted and unable to provide signals for the immune system to recognize the foreign substance. As a result, the ability of the transplanted cells to invoke immune system response (known as immunogenicity) is lost and rejection will not occur. Most importantly, these edited iPSCs are still able to retain their pluripotency and differentiation capacity. A membrane protein, CD47 is also overexpressed to inhibit phagocytosis (a process eliminating foreign substance by ingestion or engulfment). Altogether, the combination of inactivated MHC genes and overexpression of CD47 allows engineered hypoimmunogenic cells to bypass the immune system and avoid rejection. When transplanted into mice with either normal immune systems or components of human immune systems, no rejection is observed. While this is still not as close to granting us full invisibility as seen in fictions, the great potential of genome editing in granting the power of invisibility to transplant cells is nonetheless a powerful invention.

Superpower: ‘Decelerated Ageing’
Superhero: Superman
Ageing is seen as a normal part of human lives but is also commonly associated with age-related damages that can increase the risk of diseases. With ageing being a leading risk factor for debilitating diseases such as cancer, cardiovascular disease, and neurodegeneration, there is an urgent need for anti-ageing therapies to increase people’s life quality. In the fruit flies (Drosophila melanogaster), a mutation on the gene methuselah (mth) was discovered to have extended the flies’ lifespan thus earning its name based on the biblical figure Methuselah who lived up to 969 years. Interestingly, it was also found that the extended lifespan came alongside enhanced resistance to stress in mutant flies. In mammals, several genes were also discovered to prolong lifespan. With the implication of genes in ageing/anti-ageing, it is possible that genome editing can be used to target these genes to help decelerate the ageing process.
In a study done by Beyret and colleagues, CRISPR/Cas9 system was utilised to establish therapy that could suppress the accelerated ageing in mice model with a condition mimicking the Hutchinson–Gilford progeria syndrome (HGPS) seen in human. HGPS is a rare lethal genetic disorder, characterised by premature ageing beginning in childhood. This condition is the result of a mutation in the LMNA gene which normally produces proteins lamin A and lamin C. The mutation causes a shift from the production of lamin A to the toxic version of the proteins known as progerin which then drive the symptoms in HGPS when accumulated. At the same time, decreased level of lamin A can be compensated by lamin C thus, the CRISPR/Cas9 system is designed to target the LMNA gene in order to disrupt the production of lamin A/progerin. HGPS mice treated with CRISPR/Cas9 were found to have an increased lifespan by approximately 25% alongside healthier physiological functions. While treated mice still have a shorter lifespan compared to wild-type mice, the result showed promising potential for genome editing in improving life expectancy and health.
Even though solving the entire process of ageing is a little unrealistic, it looks likely that one day CRISPR could be the key to stopping a disease as common and destructive as Alzheimer’s, and we’re sure to see more from CRISPR with relation to age-associated diseases in the coming years.
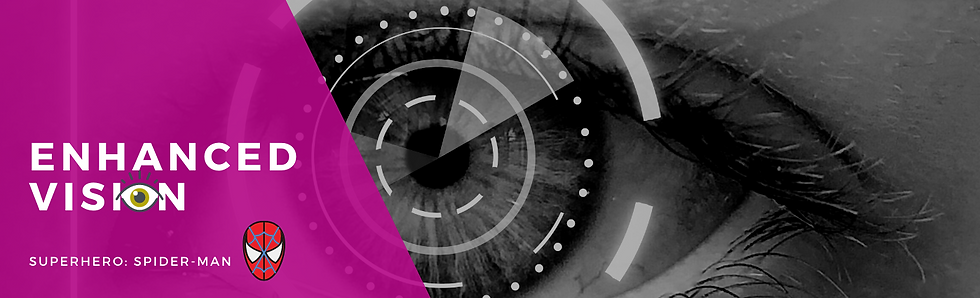
Superpower: ‘Enhanced Vision’
Superhero: Spiderman
The back of the eyes is referred to as retina, in which photoreceptors reside and convert light into signals to be sent to the brain. Two types of photoreceptors are involved in our sense of sight are they are cones and rods. While rods are useful in night vision, cones are more involved in seeing colours. The differences in the number of rods and cones determine the differences seen in human vision compared to animals. For example, jumping spiders can see ultraviolet in which most humans cannot, and birds being able to see more vividly with more photoreceptors (cones). As photoreceptors production is controlled by genes, theoretically, we can use genome editing to acquire enhanced vision- similarly to that of an eagle with 1,000,000 per square millimetre of photoreceptors (as compared to humans’ 200,000 per square millimetre). This most likely requires targeting the opsins genes found in the photoreceptors. While this has not been done in research, it is nonetheless interesting to know that enhancing vision is not that impossible.

Schematic showing photoreceptors in the retina (back of the eye) which convert light into signals to be sent to the brain. Source: CJ Kazilek& Kim Cooper (2010) "Rods and Cones" Arizona State University.
Fiction aside, a more realistic approach to enhancing vision has been done in an effort to restore vision in patients with inherited blindness. Leber’s congenital amaurosis (LCA) is a condition which affects eyesight and often manifests early in childhood. People with LCA suffer from severe vision loss as a mutation in the CEP290 gene causes deterioration of photoreceptors as time goes on. No cure has been reported for treating LCA but in 2020, mutation eradication has been attempted using CRISPR/Cas9 in a clinical trial setting. Targeting mutation on the CEP290 gene results in the increased levels of CEP290 proteins, initially observed in the mice model and in human retinal explants. Currently, the trial is still ongoing and though the result is not yet definite, this marks an exciting point in the advancement of genome editing-based therapies.
The takeaway: Genome editing technologies, especially CRISPR/Cas9 are moving fast and they do not seem to be slowing down anytime soon. With their endless potential, entertaining the possibility of gaining superpowers through genome editing is exciting but, it is probably unrealistic (at least for the near future). Regardless, scientists’ contribution to genome editing and genome editing-based therapies shows that saving people’s lives is perhaps the greatest ‘superpower’ we can ever hope to have.
…………………………………………………………………………………………………………........................
Disclaimer: The author apologises for any inaccuracies in the article, especially in relation to comic books.
References:
Alvarez, J., 2019. CRISPR Gene Editing Makes Stem Cells ‘Invisible’ to Immune System (University of California San Francisco). [Online]
Available at: https://www.ucsf.edu/news/2019/02/413311/crispr-gene-editing-makes-stem-cells-invisible-immune-system
[Accessed 31 January 2021].
Araújo, A. R. et al., 2013. The Drosophila melanogaster methuselah Gene: A Novel Gene with Ancient Functions. PLos One, 8(5), p. e63747.
Beyret, E. et al., 2019. Single-dose CRISPR–Cas9 therapy extends lifespan of mice with Hutchinson–Gilford progeria syndrome. Nature Medicine, Volume 25, p. 419–422.
Chemello, F., Bassel-Duby, R. & Olson, E. N., 2020. Correction of muscular dystrophies by CRISPR gene editing. The Journal of Clinical Investigation, 130(6), p. 2766–2776.
Deuse, T. et al., 2019. Hypoimmunogenic derivatives of induced pluripotent stem cells evade immune rejection in fully immunocompetent allogeneic recipients. Nature Biotechnology, Volume 37, p. 252–258.
Editas Medicine, 2020. Allergan And Editas Medicine Announce Dosing Of First Patient In Landmark Phase 1/2 Clinical Trial Of CRISPR Medicine AGN-151587 (EDIT-101) For The Treatment Of LCA10. [Online]
Available at: https://ir.editasmedicine.com/news-releases/news-release-details/allergan-and-editas-medicine-announce-dosing-first-patient
[Accessed 1 January 2021].
Institute, N. H. G. R., 2019. National Human Genome Research Institute. [Online]
[Accessed 29 January 2021].
Ledford, H., 2020. CRISPR treatment inserted directly into the body for first time (Nature News). [Online]
Available at: https://www.nature.com/articles/d41586-020-00655-8
[Accessed 1 February 2021].
Moreno, A. M. et al., 2019. Long-lasting Analgesia via Targeted in vivo Epigenetic Repression of Nav1.7. bioRxiv, p. 711812.
Reardon, S., 2015. Nature News. [Online]
Available at: https://www.nature.com/news/leukaemia-success-heralds-wave-of-gene-editing-therapies-1.18737
[Accessed 29 January 2021].
Santiago-Fernández, O. et al., 2019. Development of a CRISPR/Cas9-based therapy for Hutchinson–Gilford progeria syndrome. Nature Medicine, Volume 25, p. 423–426.
Terakita, A., 2005. The opsins. Genome Biology, Volume 6, p. 213.
Vega-Loza, A., Van, C., Moreno, A. M. & Aleman, F., 2020. Gene therapies to reduce chronic pain: are we there yet?. Pain Management, 10(4).
This article is prepared by Nur Farah Aida Sangkolah.
Comentarios