The Dangers of the ESKAPE Pathogens
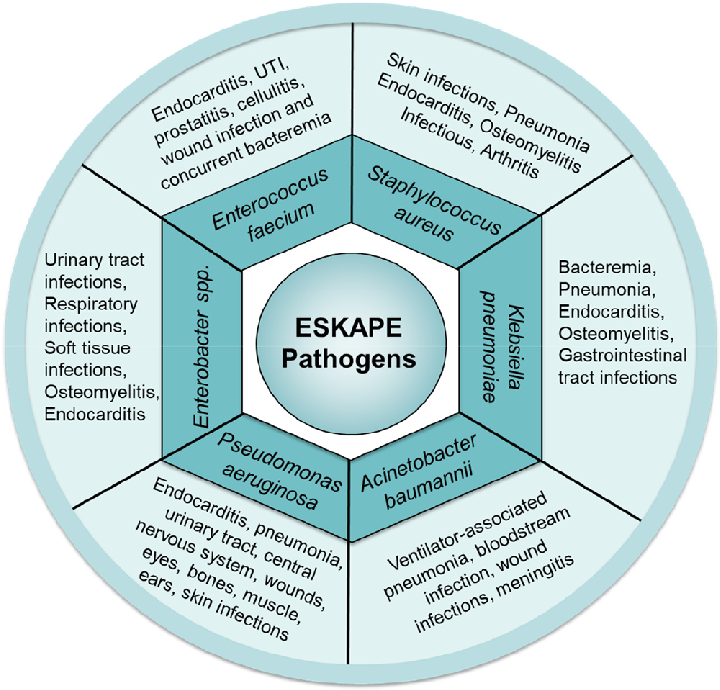
Antimicrobial-resistant ESKAPE pathogens (Enterococcus faecium, Staphylococcus aureus, Klebsiella pneumoniae, Acinetobacter baumannii, Pseudomonas aeruginosa, and Enterobacter species) pose a significant global health threat (D. M. P. De Oliveira et al., 2020). The acquisition of resistance genes by these pathogens, resulting in resistance mechanisms such as modification of a drug target, inactivation of a drug, and active efflux of a drug (Schwarz et al., 2019), has limited treatment options for serious infections, increased disease burden, and raised mortality rates due to treatment failures, necessitating a coordinated global response for antimicrobial resistance surveillance. This imminent health threat has rekindled interest in developing new antimicrobial therapies, highlighted the need for improved patient care, and prompted stricter governance over stewardship practices.
The Current State of the Antibiotic Pipeline
Of note in the ESKAPE list is that the former two aforementioned are Gram-positive microorganisms, whereas the rest are Gram-negative, highlighting that the latter has a more acute need for new antimicrobials. The importance of the ESKAPE pathogens is further highlighted by their designated ‘priority’ status by the World Health Organization (WHO) (D. M. P. De Oliveira et al., 2020). In recent years, despite the efforts, new clinically-approved antibiotics are mostly active against Gram-positive microbes, as exemplified by the synthetic oxazolidinones (linezolid), daptomycin, and pleuromutilin, but the situation is more serious for their counterparts. However, the majority of these antibiotics work by existing MOAs and only 2 new chemical classes were within this group. Since the serendipitous discovery of penicillin in 1928 that heralded the "Golden Age" of antibiotic/natural NP discovery, there has been a discovery void. The last class of clinically used NP antibiotics was lipopeptides, with a notable example of daptomycin being clinically approved for use in the US more than two decades ago (Alder, J.D., 2003). Since then, there has not been genuine novelty – in MOA and AB class – with a consecutive decline in FDA-approved antibiotics reaching the clinic and market. However, a promising contender in the war against microbes is teixobactin, a cell wall inhibitor discovered in 2015, which represents a new class of antibiotics with a unique chemical scaffold and no detectable resistance. Teixobactin targets lipid II, a precursor of peptidoglycan, disrupting cell wall synthesis and compromising the bacterial membrane by forming large supramolecular fibrils. This specific targeting of lipid II, absent in eukaryotes, mitigates toxicity concerns (Shukla, R., Lavore, F., Maity, S. et al., 2022). However, teixobactin’s selective activity against Gram-positive bacteria is likely due to its inability to reach the target in Gram-negative bacteria. This challenge could be addressed by co-administering outer membrane permeabilizers, such as polymyxins, at sub-MIC levels to enhance OM permeability and synergize with other antimicrobials like antimicrobial peptides (Venter, 2019).
As of June 2021, a total of 26 out of 76 (34%) antibacterial agents are being developed to target critical-priority WHO pathogens (carbapenem-resistant A. baumannii, carbapenem-resistant P. aeruginosa and members of the family Enterobacteriaceae which are carbapenem-resistant and containing extended spectrum β-lactamases) and 16 out of 76 (21%) are aimed at high- and medium-priority pathogens (includes methicillin-resistant, vancomycin and vancomycin-intermediate S. aureus and vancomycin-resistant E. faecium). This focus represents 55% of the overall pipeline, reflecting a positive trend in addressing key pathogens. Among traditional antibacterial agents, only two compounds—gepotidacin and zoliflodacin—are new chemical classes targeting priority pathogens. β-Lactams, with and without β-lactamase inhibitors, make up the majority of the other antibacterial agents in development against critical-priority pathogens (M. S. Butler et al., 2020).
The global clinical pipeline is dominated by derivatives of known chemical and functional classes, predominantly composed of derivatives from well-known chemical and functional classes, particularly β-lactams and β-lactamase inhibitors (M. S. Butler et al., 2020). Though the experience with widely used antibacterial classes and familiarity with class-specific safety profiles and pharmacokinetic and pharmacodynamic properties are good starting points for antibacterial research and development programmes, chemical modifications lead to improvements that are usually incremental and address only selected class-specific resistance mechanisms that are known at the time of lead optimization, whereas other mechanisms remain unaffected. Thus, a relatively high rate of pre-existing cross-resistance in XDR strains or substantially increased minimum inhibitory concentrations compared with the wild-type strains may limit the benefit of such new therapies in many geographic regions and locations (Theuretzbacher, U., (2023)). Furthermore, while some resistance mechanisms specific to these classes are being addressed, others remain unaddressed, highlighting the need for continued innovation in antimicrobial drug development (M. S. Butler et al., 2020).
Conclusion
Despite numerous alarming headlines, multidrug resistance remains relatively rare and predominantly affects low- and middle-income countries, where factors such as limited access to newer drugs and inadequate sanitation exacerbate the issue (Ranjbar, R. and Alam, M., (2023)). As antimicrobial resistance is a natural phenomenon, resistance levels are expected to increase over time. However, as aforementioned, this issue is more pronounced with Gram-negative bacteria due to the limited development of new antibiotics for these pathogens. To prolong the effectiveness of antibiotics and combat the rise of resistance, it is crucial to implement strategies such as reducing unnecessary antibiotic prescriptions, inhibiting mutations or horizontal gene transfers that confer resistance, and exploring alternative methods.
Article prepared by: Melisa Wong Siang Ming, MBIOS R&D Associate 23/24
If you enjoyed this article, do sign up to become a part of our MBIOS family and receive our monthly newsletter along with many more resources in the link below.
References
Alder, J.D. (2003) Daptomycin, a new drug class for the treatment of Gram-positive infections. Drugs of Today v. 41, n. 2 (2005): 81. Available from: 10.1358/dot.2005.41.2.882660
Butler, M.S., Gigante, V., Sati, H., Paulin, S., et al. (2022) Analysis of the Clinical Pipeline of Treatments for Drug-Resistant Bacterial Infections: Despite Progress, More Action Is Needed. Antimicrobial Agents and Chemotherapy. [Online] 66 (3). Available from: doi:10.1128/aac.01991-21.
De Oliveira, D.M.P., Forde, B.M., Kidd, T.J., Harris, P.N.A., et al. (2020) Antimicrobial Resistance in ESKAPE Pathogens. Clinical Microbiology Reviews. [Online] 33 (3). Available from: doi:10.1128/cmr.00181-19.
Ranjbar, R. and Alam, M. (2023) ‘Antimicrobial resistance collaborators (2022). global burden of bacterial antimicrobial resistance in 2019: A systematic analysis’, Evidence Based Nursing, 27(1), pp. 16–16. doi:10.1136/ebnurs-2022-103540.
Shukla, R., Lavore, F., Maity, S. et al. Teixobactin kills bacteria by a two-pronged attack on the cell envelope. Nature 608, 390–396 (2022). Available from: doi.org/10.1038/s41586-022-05019-y
Schwarz, S., Cloeckaert, A. and Roberts, M.C. (2019) ‘Mechanisms and spread of bacterial resistance to antimicrobial agents’, Antimicrobial Resistance in Bacteria of Animal Origin, pp. 73–98. doi:10.1128/9781555817534.ch6.
Theuretzbacher, U. (2023) ‘Evaluating the innovative potential of the global antibacterial pipeline’, Clinical Microbiology and Infection [Preprint]. doi:10.1016/j.cmi.2023.09.024.
Venter, H. (2019b) ‘Reversing resistance to counter antimicrobial resistance in the World Health Organisation’s critical priority of most dangerous pathogens’, Bioscience Reports, 39(4). doi:10.1042/bsr20180474.
Comments