Monday Article #54: Tackling Vancomycin’s Ineffectiveness Against Gram-Negative Bacteria
Discovered in the 1950s, vancomycin, a glycopeptide prototype, has been used as treatment for Gram-positive bacteria such as enterococci and staphylococci. However, due to the nature of evolution, there has been a rise in vancomycin-resistant enterococci and staphylococci (Stogios and Savchenko, 2020). As vancomycin is clinically effective against most gram-positive bacteria, it has been known to have no effect on gram-negative bacteria. Vancomycin, like other glycopeptide targeted antibiotics, works by binding onto the D-Ala-D-Ala termini found on the peptidoglycan layer (Antonoplis et al., 2019). Due to the presence of the liposaccharide (LPS) outer membrane only found in Gram-negative bacteria, as shown in Figure 1. (Sun et al., 2022), it prevents the binding of vancomycin.

The LPS membrane acts as a barrier that prevents pathogenic substances from affecting the bacterial cell, such that it is ineffective against hydrophobic antibiotics that are generally effective against Gram-positive bacteria, and large molecules (Sun et al., 2022). Thus, this makes Vancomycin unable to penetrate through the outer membrane and trigger bactericidal activities (Antonoplis et al., 2019).
Modifying molecular structure of vancomycin
Modification of the chemical structure of vancomycin has been shown to have increased its effectiveness against Gram-negative bacteria. Research had found an increased activity against Gram-negative bacteria when an arginine was covalently attached to vancomycin as shown in Figure 2. With respect to Enterococci coli, 2-arginine and a single arginine conjugate was equally more successful than vancomycin, however the latter was effective against other other Gram-negative bacteria such as V. cholerae, A. baumannii, and P. aeruginosa. Arginine could not generate antibacterial activity, thus requiring the covalent conjugation to vancomycin (Antonoplis et al., 2019).
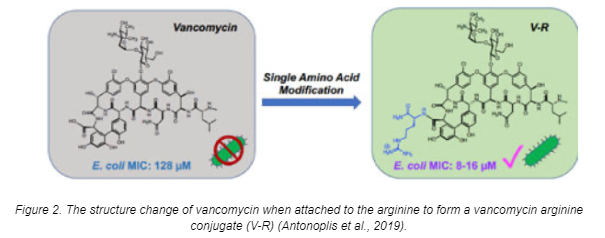
The research had also identified the reasoning behind the increased effectiveness. Due to the positively charged amino acid bonded to vancomycin, it increases its electrostatic attraction to the negatively charged LPS. Additionally, the mechanism leading to a declining bacterial cell growth was evaluated. Since vancomycin has been associated with the loss of morphology in E. coli, the disruption in peptidoglycan synthesis was consistent when E. coli was treated with vancomycin and V-R at the same concentration, though V-R was evidently more effective (Antonoplis et al., 2019).
Utilisation of 1-N-phenylnaphthylamine (NPN) allows the permeability of the outer membrane to be assessed. Following its uptake upon treatment of V-R, it shows consistency with other antibiotics that promote cellular uptake. These antibiotics are able to compete with Mg2+ in its cation channels and enter the cell. Therefore, this simple chemical modification of vancomycin is able to trigger mechanisms that lead to morphological changes and a declining bacterial growth, suggesting its use in therapeutics against Gram-negative bacteria (Antonoplis et al., 2019).
In an attempt to further make Gram-negative bacteria more susceptible to vancomycin, another researcher has designed a conjugation that would allow the penetration of the LPS outer membrane by vancomycin-derived antibiotic. This included the connection between vancomycin and polymyxin E nonapeptide (PMEN), which is referred to as vancomyxin, refer to Figure 3. For the structure. The vancomyxin showed an increased efficacy against Gram-negative bacteria in comparison to vancomycin and vancomycin and PMEN administered separately. Although effectiveness of separately administered vancomycin and PMEN is consistent with previous discoveries, the covalent linkage between PMEN and vancomycin that produces vancomyxin had a stronger effect against most strains that were used in the experiment, notably E. coli (van Groesen et al.,2021). The commonality between these two modified vancomycin was noticed that covalent bonding at its C-termini has the greatest effect against Gram-negative strains.

Altering the physical structure of Vancomycin
An alternative method of increasing the susceptibility of Gram-negative bacteria is sizing down Vancomycin. The use of nanotechnology is a relatively new area in biotherapeutics, thus the concepts are not fully understood. Properties of nanosized particles differ from those of free molecules of the same type. Transforming antimicrobial agents into nanospheres alters its physical properties, thus allowing action against microbes. Reports mention antibacterial nanospheres are more effective against bacteria compared to its regular counterpart. The mechanisms that were reported to have associated with the treatment of nanosized antibacterial, such as membrane permeability, osmotic damage, and outflow of cytoplasmic components, decreases the likelihood of the development of resistant strains (Fernandes et al., 2017).
Vancomycin nanospheres were used against E. coli and P. aeruginosa strains and found a 10 and 100 times increase in susceptibility respectively. The impermeability of the double layered Gram-negative bacteria to large glycopeptide molecules, such as vancomycin, restricts the access of the target that leads to bacterial death. Nanosphere stabilisation was supported by a hydrogen-bond network that was created upon the formation of nanosized vancomycin. Furthermore, the nanosized vancomycin has a larger surface area, which strengthens the membrane disruption and cell leakage caused by the attraction between the positively charged nanospheres and negatively charged membrane (Fernandes et al., 2017).
As seen in Figure 4., the shape of E. coli differed after being treated with nanosized vancomycin. The typical shape of E. coli is rod-like and smooth surface, whereas the morphed shaped indicates the presence of cell damage (Fernandes et al., 2017).
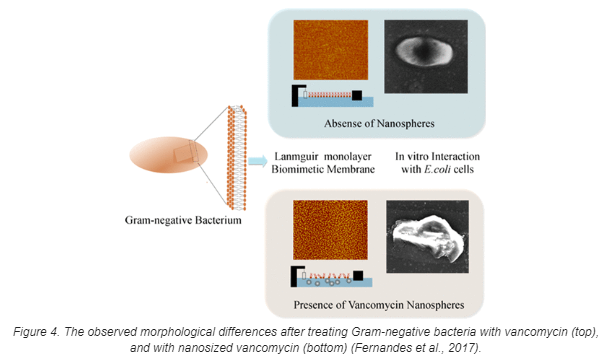
Conclusion
Although Gram-negative bacteria are intrinsic resistant to glycopeptide antibacterial molecules, there are other methods that could tackle the resistance through chemical modification and the use of technology. Furthermore, research has open doors to non-antibacterial therapeutic technologies such as the use of monoclonal antibodies.
Reference(s)
Antonoplis, A. et al. (2019) “Vancomycin–arginine conjugate inhibits growth of carbapenem-resistant E. coli and targets cell-wall synthesis,” ACS Chemical Biology, 14(9), pp. 2065–2070. Available at: https://doi.org/10.1021/acschembio.9b00565.
Fernandes, M.M. et al. (2017) “Nanotransformation of vancomycin overcomes the intrinsic resistance of gram-negative bacteria,” ACS Applied Materials & Interfaces, 9(17), pp. 15022–15030. Available at: https://doi.org/10.1021/acsami.7b00217.
Stogios, P.J. and Savchenko, A. (2020) “Molecular mechanisms of vancomycin resistance,” Protein Science, 29(3), pp. 654–669. Available at: https://doi.org/10.1002/pro.3819.
Sun, J., Rutherford, S.T., Silhavy, T.J. and Huang, K.C., 2022. Physical properties of the bacterial outer membrane. Nature Reviews Microbiology, 20(4), pp.236-248.
van Groesen, E. et al. (2021) “Vancomyxins: Vancomycin-polymyxin nonapeptide conjugates that retain anti-gram-positive activity with enhanced potency against gram-negative strains,” ACS Infectious Diseases, 7(9), pp. 2746–2754. Available at: https://doi.org/10.1021/acsinfecdis.1c00318.
This article was prepared by Fatini Khadrishah
Comments